19 June 2023–Interested in math and science early on, and looking for a job that would benefit society, Greg McLaskey went to college to study civil engineering.
“The thing that excited me the most about civil engineering was non-destructive testing,” he recalled, so he was soon learning all about wave propagation and signal analysis for concrete buildings, with seismology courses as part of his minor.
Seismology continued to beckon, said McLaskey, now an associate professor of civil and environmental engineering at Cornell University. In graduate school for civil engineering at University of California, Berkeley, he worked on a method called acoustic emission. “If there’s a crack in something, it causes little vibrations. And I always thought of them as little baby earthquakes,” he explained. And then there was a course in active tectonics with UC Berkeley’s Roland Bürgmann, and then three years working at the U.S. Geological Survey in Menlo Park.
“I realized that I’m not just interested in this from a civil engineering perspective,” he said, “I’m interested in it because earthquakes are amazing, and I want to know more.”
Today McLaskey works in the field of earthquake mechanics, studying how earthquakes begin, the physics and mechanics of how an earthquake rupture propagates, and a question that might seem unusual at first glance: how do earthquakes stop?
“The prevailing view of how earthquakes work is that they just keep rupturing, and the big ones that we feel are just the ones that don’t stop,” he said. “So a magnitude 1 is just a magnitude 8 that happened to stop prematurely.”
The mechanisms by which earthquakes stop help control the size distribution of earthquakes, McLaskey noted. “If you want to know why some earthquakes are big or if there’s a potential for an earthquake to be really big on a fault, then you need to know what could potentially stop that rupture, or if those barriers that could stop the rupture are permanent or impermanent.”
Recorded seismic data offers “kind of a blurry view” at the end of an earthquake, and numerical modeling rarely considers this phenomenon, said McLaskey. To learn more about earthquake stopping, he studies simulated earthquakes with one of the largest simulation configurations available.
Using a machine he designed and built himself, he and his students can induce earthquake loading on a slab of granite 3 meters long and 1.5 meters wide—far larger than the usual “tabletop” earthquake set-up. “Multiple people can be sitting on top of it, gluing sensors to it,” McLaskey said.
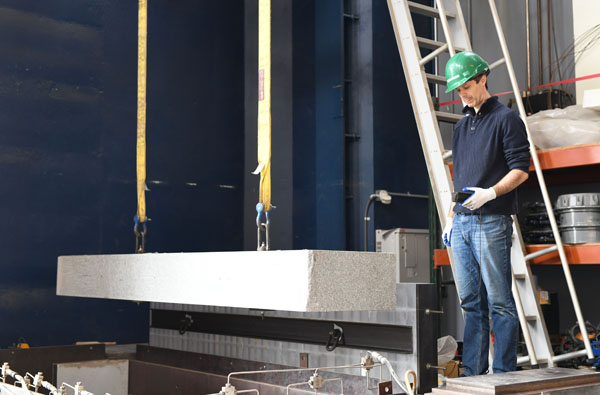
Size matters when it comes to understanding how a simulated earthquake stops, he explained. “In the lab if you have a small sample, there’s no way to get it so that only part of the sample ruptures in the experiment, it usually consumes the entire sample.”
At the USGS and now at Cornell, however, “we’ve been working on experiments where it’s really possible to generate an earthquake-like rupture in the lab that stops in the middle of the sample,” he said.
“The thing that’s been really unexpected that I’ve learned over the past 10 years or so is that you think these are like flat surfaces,” McLaskey said. “So you expect the stress conditions to be uniform across the sample, and they’re really not. There are really huge differences in the normal stress or the sheer stress from one part of the sample to another.”
Measuring these differences can be difficult, even in a large sample. One tactic his lab has used is digital image correlation. By painting speckles on samples and taking pictures of the sample before and after earthquake loading, they can track how those speckles were deformed. The trick then is to apply these findings to how deformation might take place along a real-life fault.
Seven or eight years ago, he said, his dream was to create a fully contained dynamic rupture in the lab. Now that he and his colleagues have accomplished that, “the next thing I would like to do is generate an aftershock sequence in the lab.
“We’re trying to build the tools to do that and figure out how to make a ‘bump’ on a fault that will generate seismicity in a controlled way. We have a sample big enough so that those ‘bumps’ can rupture independently and interact,” McLaskey noted, bringing the simulations closer to natural earthquake mechanisms.
The seismology community has welcomed him as he works less on concrete buildings and more on earthquake mechanics, he said. “My advice is not to work in isolation. Just from knocking on doors at USGS, I learned so much. And this is a great community for sharing data, so take advantage of that.”
SSA At Work is a monthly column that follows the careers of SSA members. For the full list of issues, head to our At Work page.